Introduction: Magnesium oxide (MgO) has become increasingly attractive for biomedical applications, particularly bone repair, due to its bioactivity[1]-[4]. Clinically, MgO has been taken as an oral supplement to improve bone mineral density[5]. More recently, MgO powder paste was shown to increase the thickness in compact bone of the rat tibia[6]. These properties make MgO a promising material for treating bone-related trauma and diseases.
Hydroxyapatite (HA) is a naturally occurring form of calcium phosphate. It is widely favored as an ideal material for use in bone regeneration as it has been shown to be osteoconductive and osteoinductive[7]. We chose to evaluate HA alongside MgO for comparison to a clinically viable material.
We developed a composite of poly(lactic-co-glycolic acid) (PLGA) with either MgO or HA, using exclusively mechanical dispersion. These composites were characterized via optical methods and cytocompatibility was evaluated using bone marrow stromal cells (BMSCs).
Materials and Methods: MgO nanoparticles, were purchased from US Research Nanomaterials Inc. (20nm, US3310). Hydroxyapatite was synthesized through wet-precipitation using well established protocols in our lab[8].
PLGA (50:50, MW 100,000 Da) was purchased from Polyscitech. Well dispersed PLGA/MgO and PLGA/HA composites were synthesized through solvent casting and nanoparticles were dispersed using mechanical stimulation. Composites with agglomeration did not undergo the same mechanical stimulation, resulting in particle agglomeration.
Composites and nanoparticles were characterized through scanning electron microscopy (SEM), optical transparency, and fourier transform infrared spectrometry (FTIR).
Rat BMSCs at the second passage were incubated under standard cell culture conditions with the nanocomposites for 24 hours.
Results and Discussion:
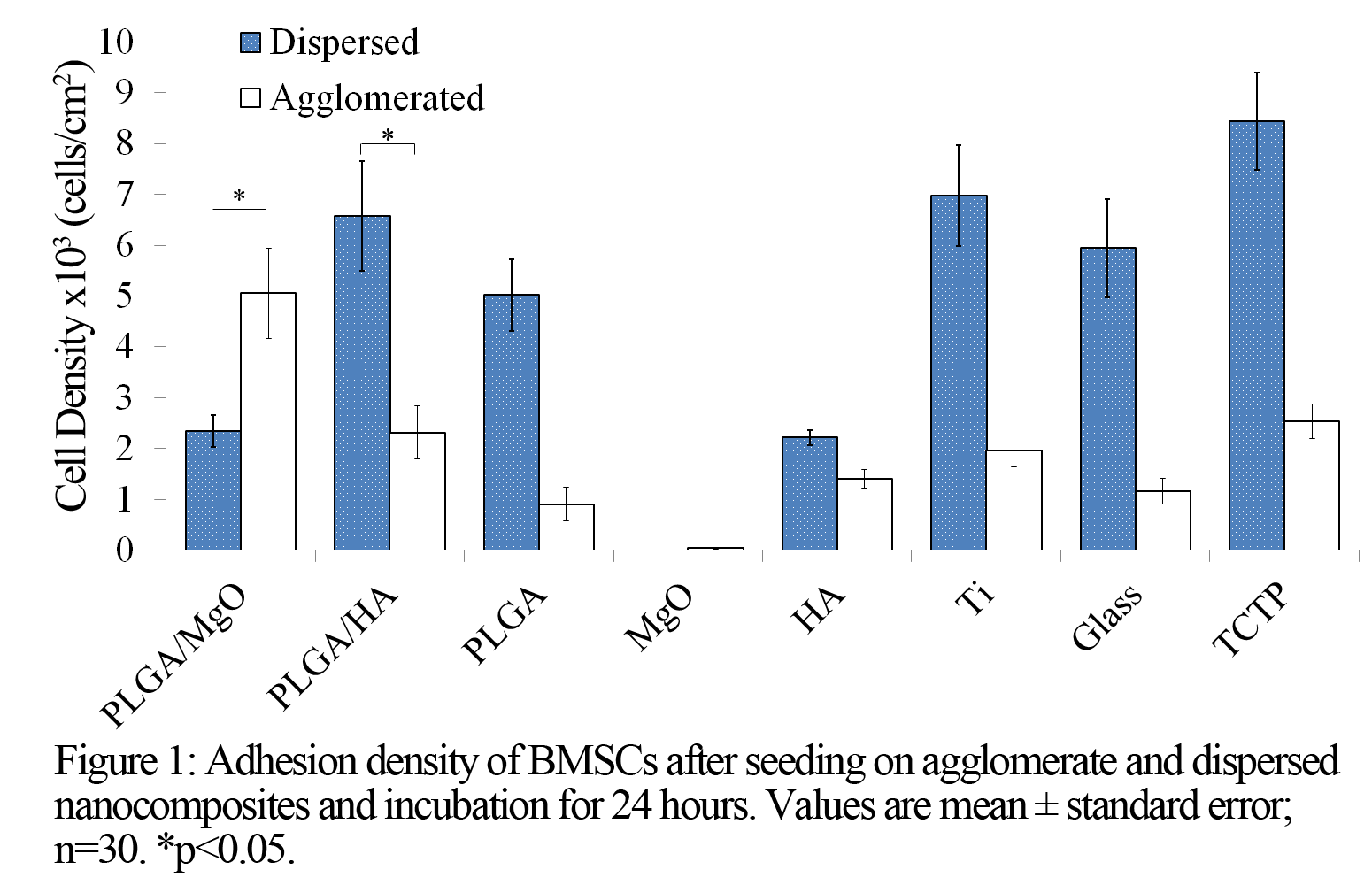
Transmission values in the visible spectrum confirmed that dispersed PLGA/MgO and PLGA/HA are more optically transparent than their agglomerated counterparts. Agglomerated PLGA/MgO showed statistically higher cell density compared to the tissue culture treated plate (TCTP) control (Figure 1). Dispersed PLGA/MgO showed statistically lower cell density compared to the TCTP control and the agglomerated PLGA/MgO. The higher cell density on agglomerated PLGA/MgO may be from the decreased cell-MgO contact due to reduced surface area of MgO. Conversely, the increased cell-MgO contact in dispersed PLGA/MgO resulted in toxic MgO levels. In contrast, dispersed PLGA/HA showed statistically higher cell density compared to agglomerated PLGA/HA, showing enhanced cell adhesion from better dispersion, which is in agreement with literature[9].
Conclusion: Our mechanical stimulation methods improved HA and MgO particle dispersion in PLGA. Indirect contact with MgO results in improved BMSC adhesion. Improved dispersion of HA also results in improved BMSC adhesion. Further development of composites using slower degrading polymers with less MgO is necessary to improve the biocompatibility of MgO containing nanocomposites.
U.S. National Science Foundation; Burroughs Wellcome Fund; Hellman Faculty Fellowship; University of California (UC) Regents Faculty Fellowship; Central Facility for Advanced Microscopy and Microanalysis at UC Riverside
References:
[1] Fielding, G.A., W. Smoot, and S. Bose, Effects of SiO2, SrO, MgO, and ZnO dopants in tricalcium phosphates on osteoblastic Runx2 expression. J Biomed Mater Res A, 2014. 102(7): p. 2417-26.
[2] Kum, C.H., et al., A poly(lactide) stereocomplex structure with modified magnesium oxide and its effects in enhancing the mechanical properties and suppressing inflammation. Small, 2014. 10(18): p. 3783-94.
[3] Pourdanesh, F., et al., In vitro and in vivo evaluation of a new nanocomposite, containing high density polyethylene, tricalcium phosphate, hydroxyapatite, and magnesium oxide nanoparticles. Mater Sci Eng C Mater Biol Appl, 2014. 40: p. 382-8.
[4] Tarafder, S., et al., SrO- and MgO-doped microwave sintered 3D printed tricalcium phosphate scaffolds: Mechanical properties and in vivo osteogenesis in a rabbit model. J Biomed Mater Res B Appl Biomater, 2014.
[5] Carpenter, T.O., et al., A randomized controlled study of effects of dietary magnesium oxide supplementation on bone mineral content in healthy girls. J Clin Endocrinol Metab, 2006. 91(12): p. 4866-72.
[6] Nygren, H., et al., Increase of Compact Bone Thickness in Rat Tibia after Implanting MgO into the Bone Marrow Cavity. J Funct Biomater, 2014. 5(3): p. 158-66.
[7] Venkatesan, J. and S.K. Kim, Nano-hydroxyapatite composite biomaterials for bone tissue engineering--a review. J Biomed Nanotechnol, 2014. 10(10): p. 3124-40.
[8] Johnson, I., K. Akari, and H. Liu, Nanostructured hydroxyapatite/poly(lactic-co-glycolic acid) composite coating for controlling magnesium degradation in simulated body fluid. Nanotechnology, 2013. 24(37): p. 375103.
[9] Liu, H., E.B. Slamovich, and T.J. Webster, Increased Osteoblast Functions on (Poly-lactic-co-glycolic acid) with Highly Dispersed Nanophase Titania Journal of Biomedical Nanotechnology, 2005. 1(1) p. 83-89