Introduction: Lung cancer represents the leading cause of cancer-related death and continues to have the highest new cases in the United States[1]. An accurate model that mimics the microenvironment of tumor could promote the discovery of new drugs and effective treatments of lung cancer. Cancer cells cultured within a three-dimensional (3D) environment behave significantly different in proliferation and signal pathway compared with two-dimensional (2D) substrates[2]. Furthermore, 3D models closely simulate the physiological and pathological conditions of animal models, allowing inexpensive studies in vitro. This study aimed to develop a decellularized lung extracellular matrix (DL-ECM) hydrogel as a 3D lung cancer model.
Materials and Methods: Adult porcine lungs were collected from a local slaughterhouse and stored at -80 oC. The frozen lungs were sliced and decellularized using 0.5% sodium dodecyl sulfate (SDS) for 3 d followed by thorough resining with deionized water and then lyophilized to obtain DL-ECM. The DL-ECM hydrogel was prepared using an enzymatic digestion method as ref[3],[4]. Briefly, DL-ECM was digested with pepsin in 0.01 M HCl at ECM/pepsin ratio of 10/1 for 2-3 days, until no visible particle was found. The digest was neutralized with 0.1 M NaOH and 10X PBS to obtain pre-gel solution, which was then diluted into pre-determined ECM concentrations and solidified at 37 oC for 30 min to form hydrogels.
The DL-ECM hydrogels were characterized in morphologies, gelation behaviors and compressive mechanical properties using scanning electron microscope (SEM), turbidimetric absorbance and MTS Insight machine, respectively. The biocompatibility was assessed by seeding the hydrogel with A549 cells. The cells were mixed with the pre-gel solution at the cell density of 1×107 cells/mL and induced to hydrogels at 37 oC, and then maintained in an incubator with 5% CO2 and 95% humidity. Live/dead staining was performed 3 d after seeding to demonstrate cell viability.
Results and Discussion: The DL-ECM had the major component of collagen and a minor component of glycosaminoglycan (data not shown) and was processed into hydrogels. SEM image showed that the hydrogel had a porous, fibrous structure with good interconnection (Fig.1A). The gelation curves indicated that the hydrogel could firmly form within 30 min, and the gelation behavior was independent of ECM concentration (Fig. 1B). The peak compressions of the hydrogels increased from 162 ±12 to 698 ± 26 Pa as the ECM concentration increased from 9 to 18 mg/mL (Fig. 1C). It was notable that higher ECM concentrations (15 and 18 mg/mL) resulted in significantly greater peak compressions. The mechanical properties of hydrogels could be easily altered by varying ECM concentration, which is desirable to create a microenvironment mechanically similar to lung tumor and to modulate cell-matrix interactions.
A549 cells were mixed with pre-gel solution and evenly distributed in the hydrogel (Fig. 1D). It was observed that the A549 cells exhibited circular morphology with predominate live cells (green dots) throughout the hydrogel after 3 d, indicating a high survival rate. Therefore, the DL-ECM hydrogel was highly biocompatible and might provide a good platform for cancer cell proliferation and tumor formation in vitro. In addition, the ECM hydrogel could be delivered into body by injection, which might efficiently fill the gaps between in vitro and in vivo studies [4].
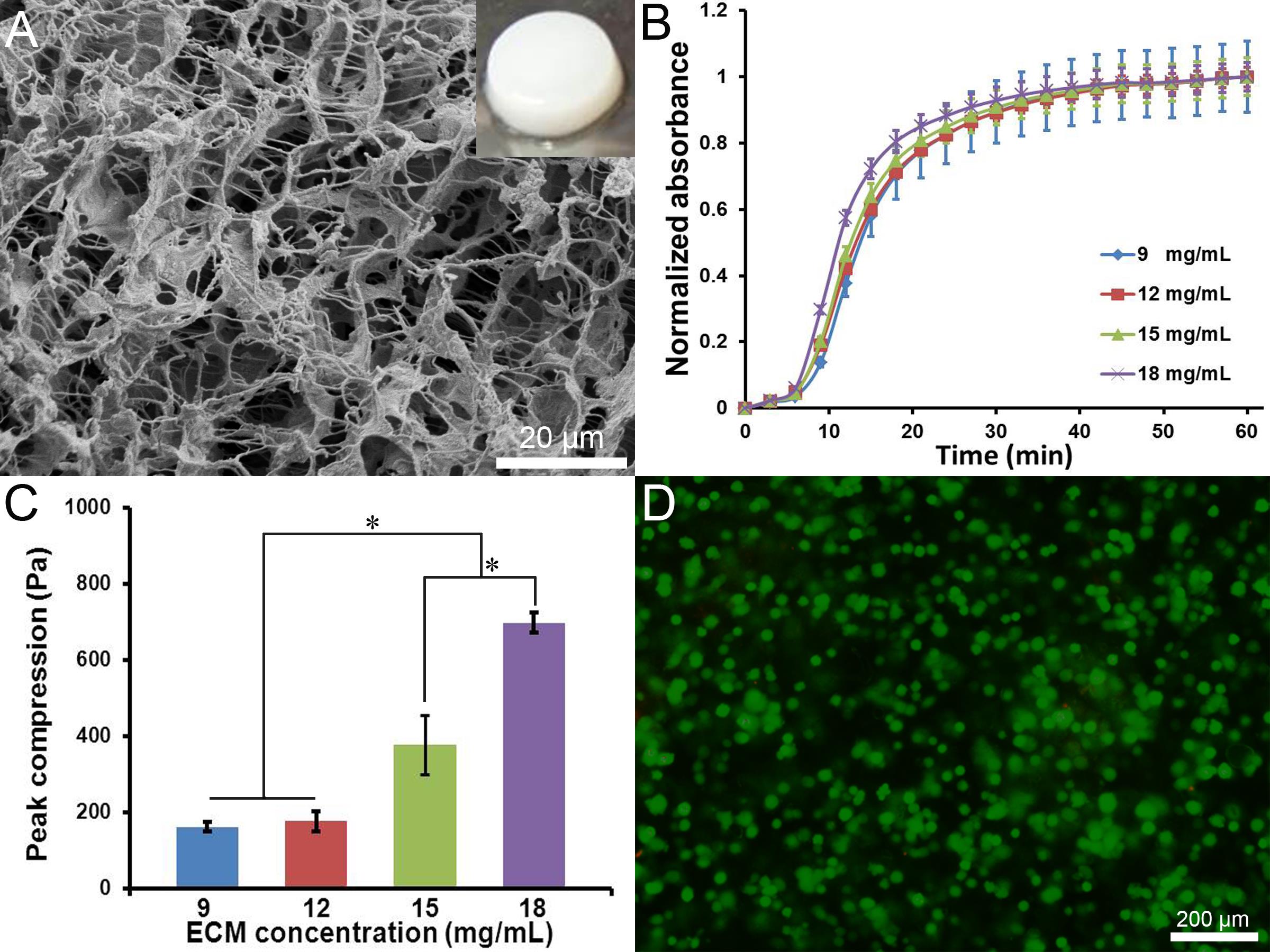
Figure 1. (A) SEM image of the DL-ECM hydrogel (insert is the macroscopic image). (B) gelation curves and (C) compressive stresses of the hydrogels at different ECM concentrations. (D) live/dead staining of A549 cells encapsulated in the hydrogel for 3d.
Conclusions: We developed a DL-ECM hydrogel and elucidated its excellent characteristics that were biochemically similar to native ECM and mechanically tunable. The hydrogel was biocompatible to support lung cancer cell growth. These findings provided proof-of-principle for further study of using the hydrogel as a 3D model to explore effective treatments for lung cancer diseases.
Startup grant of the University of Texas at Arlington
References:
[1] Siegel R, et al. CA Cancer J Clin 2014;64:9-29
[2] Herrmann D, et al. Carcinogenesis 2014;35:1671-9
[3] Jeffords ME, et al. ACS Appl Mater Interfaces 2015;7:11053-61
[4] Wu J, et al. Acta Biomater 2015;16:49-59