Introduction: Scaffold plays important role in tissue engineering, which can provide a 3D environment for cells growth and guide tissue regeneration. In recent years, nanofibrous scaffold began to be fabricated which can mimic the structure of the nature extracellular matrix (ECM) 1. There are mainly three processing techniques (electrospinning, self-assembly, and phase separation) for preparing nanofibrous scaffold[1],[2]. In this study, we prepare a 3D nanofibrous scaffold by electrospinning and freeze-drying technique. In contrast to many other scaffolds, this scaffold showed nanofibrous structure and superelastic propertie. Cells culture study showed that the scaffold offered a proper microenvironment for cells growth.
Materials: Gelatin was purchased from MP Biomedicals, LLC. PLA was purchased from Jinan Daigang Biomaterial Co., Ltd. All other chemicals were of analytical grade and commercially available.
Methods: Gelatin and PLA were dissolved in 1,1,1,3,3,3-Hexafluoro-2-propanol (HFIP), the total concentration of gelation and PLA were 11% (W/V). Then the solution was used for electrospinning. After electrospinning, the electrospun gelatin/PLA nanofibers membranes were cut into small pieces and dispersed in tert-butanol by homogenizer, then the dispersions were frozen and freeze dried. Scaffold was crosslinked by glutaraldehyde or EDC/NHS. At last, the scaffold was washed by water several times for using. The scaffolds were gold sputtered and observed under SEM. Compression tests were carried out by Instron 5969 testing system. The proliferation of cells after cultured on the scaffold 6 days was evaluated by laser scanning confocal microscope.
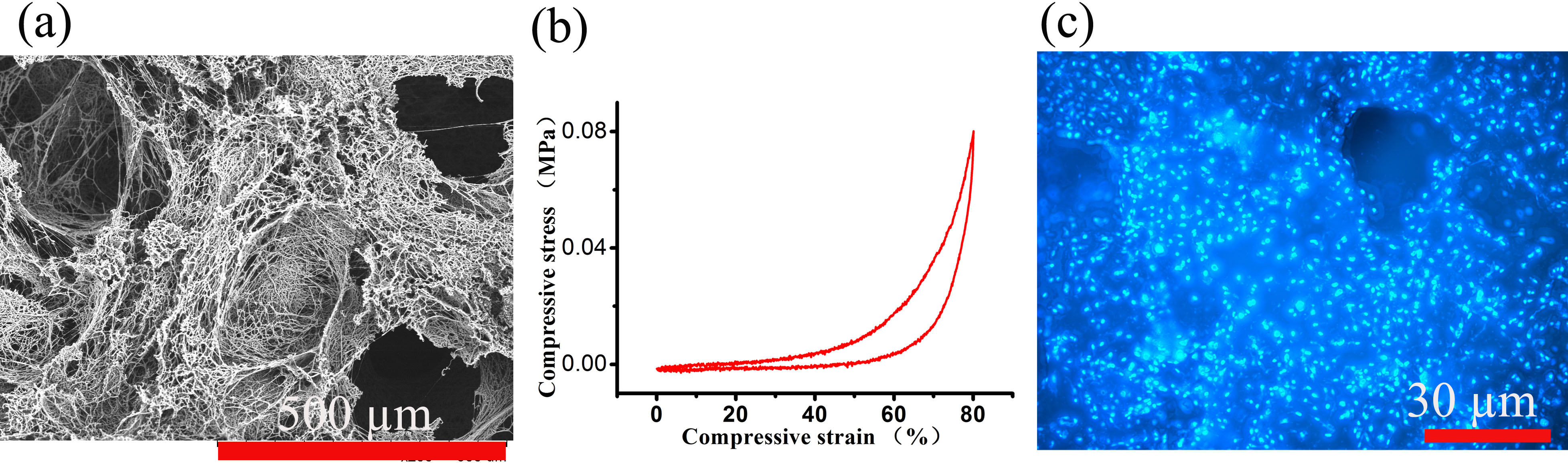
Fig. 1 (a) SEM image of 3D scaffold, (b) Compressive mechanical property of the scaffold in wet state, (c) Confocal image of L-929 cells cultured on 3D scaffold.
Results and Discussions: Fig.1 (a) showed the SEM image of scaffold, nanofibers and pores could be found. Fig. 1 (b) showed the compressive stress-strain curves of the scaffold in wet state. The scaffold could bear a compressive strain as high as 80%. Nanofibers with strong bonding were formed after crosslink, so it could recover their original shape after the stress was released. Fig. 1 (c) showed the confocal image of L-929 cultured on scaffold. It could be observed that cells attached and spread to the surface of scaffold. Scaffold might provided enough three dimensional space with porous structure for cell proliferation.
Conclusions: The scaffold presented porous morphology. In wet state, the scaffold also showed superelastic property and could bear a compressive strain as high as 80%. This scaffold could enhance cell growth and proliferation. It might be a promising scaffold for tissue engineering.
References:
[1] J.M. Holzwarth and P.X. Ma, 3D nanofibrous scaffolds for tissue engineering, Journal of Materials Chemistry, 21 (2011) 10243-10251.
[2] X. Wang, B. Ding and B. Li, Biomimetic electrospun nanofibrous structures for tissue engineering, Materials Today, 16 (2013) 229-241.