Introduction: The use of decellularized tissues in regenerative medicine is a promising approach due to the preservation of complex constituents and architecture of the native extracellular matrix (ECM). The tissue-specific ECM is crucial in regulating cellular behaviour and harnessing this innate function may be advantageous in strategies to direct cell proliferation, differentiation, and migration[1]. In this work, decellularized adipose tissue, dermis, or myocardium was used to fabricate non-chemically crosslinked porous microcarriers as cell expansion and injectable cell delivery vehicles for tissue-specific soft connective tissue regeneration.
Materials and Methods: Decellularized human adipose tissue (DAT), porcine dermis (DDT), and porcine left ventricular myocardium (DLV) were prepared following established protocols (REB# CHEM-002-07)[2]-[4]. The tissue was enzymatically digested with α-amylase and homogenized in 0.2 M acetic acid to generate an ECM suspension. The microcarriers were fabricated by electrospraying the suspension (15, 25, and 35 mg/mL) into liquid nitrogen, followed by lyophilisation[2]. The size distribution was assessed, and the ECM composition and microarchitecture were characterized using IHC (collagen I, collagen IV, laminin, and fibronectin) and SEM. To assess the potential of the ECM-derived microcarriers as a tissue-specific cell culture and delivery platform, human adipose-derived stem cells (ASCs) were seeded onto the DAT microcarriers and dynamically cultured within a CELLSPIN system. Cell attachment, proliferation, and infiltration were monitored using Live/Dead® staining, Guava ViaCount Assay, and DAPI staining over 1 month in comparison to commercial Cultispher-S microcarriers, and the ASC immunophenotype was confirmed by flow cytometry. Viability of the ASCs seeded on the DAT microcarriers was also assessed following injection through a needle and after cryo-preservation.
Results and Discussion: The DAT, DDT, and DLV microcarriers were structurally robust in culture over 1 month without the need for chemical crosslinking. SEM revealed a porous spherical morphology.
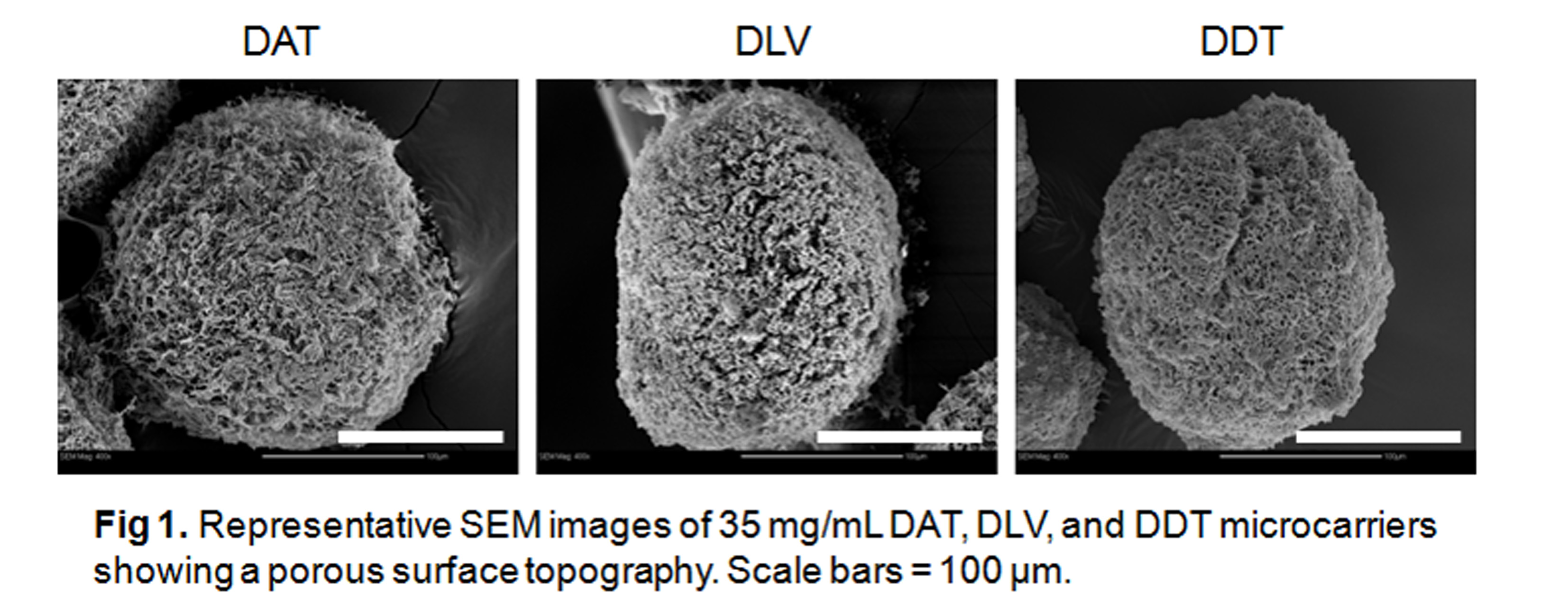
IHC confirmed the preservation of collagen I and IV, fibronectin, and laminin in the DDT microcarriers, while only collagen I and IV were detected in the DAT and DLV microcarriers.
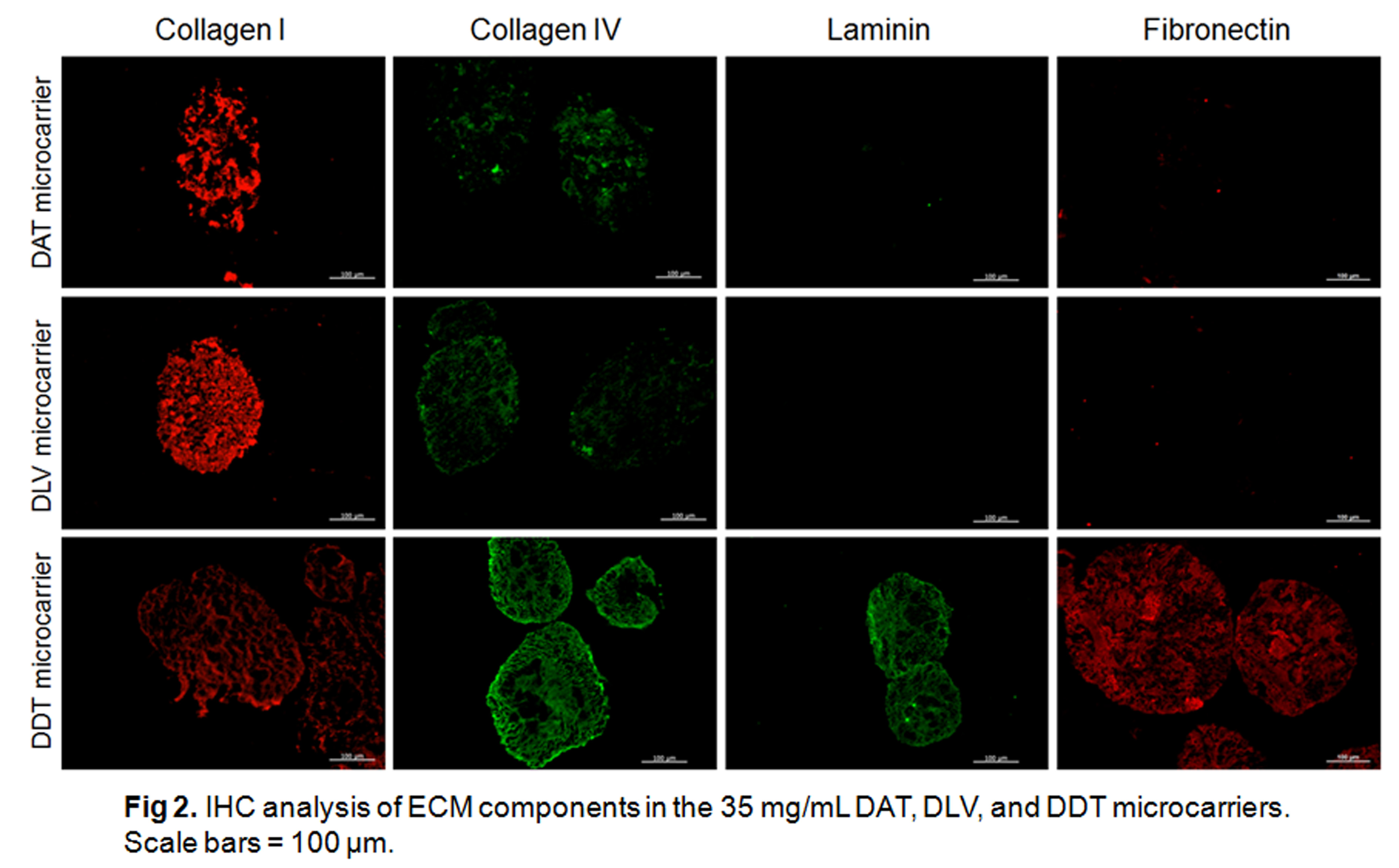
Culture studies revealed enhanced ASC attachment and proliferation on the DAT microcarriers as compared to Cultispher-S microcarriers, with cell infiltration observed at 3 and 4 weeks. The injectability and ability to freeze the cell-seeded microcarriers may be advantageous in the translation of the technology as a minimally-invasive cell delivery platform for soft connective tissue regeneration.
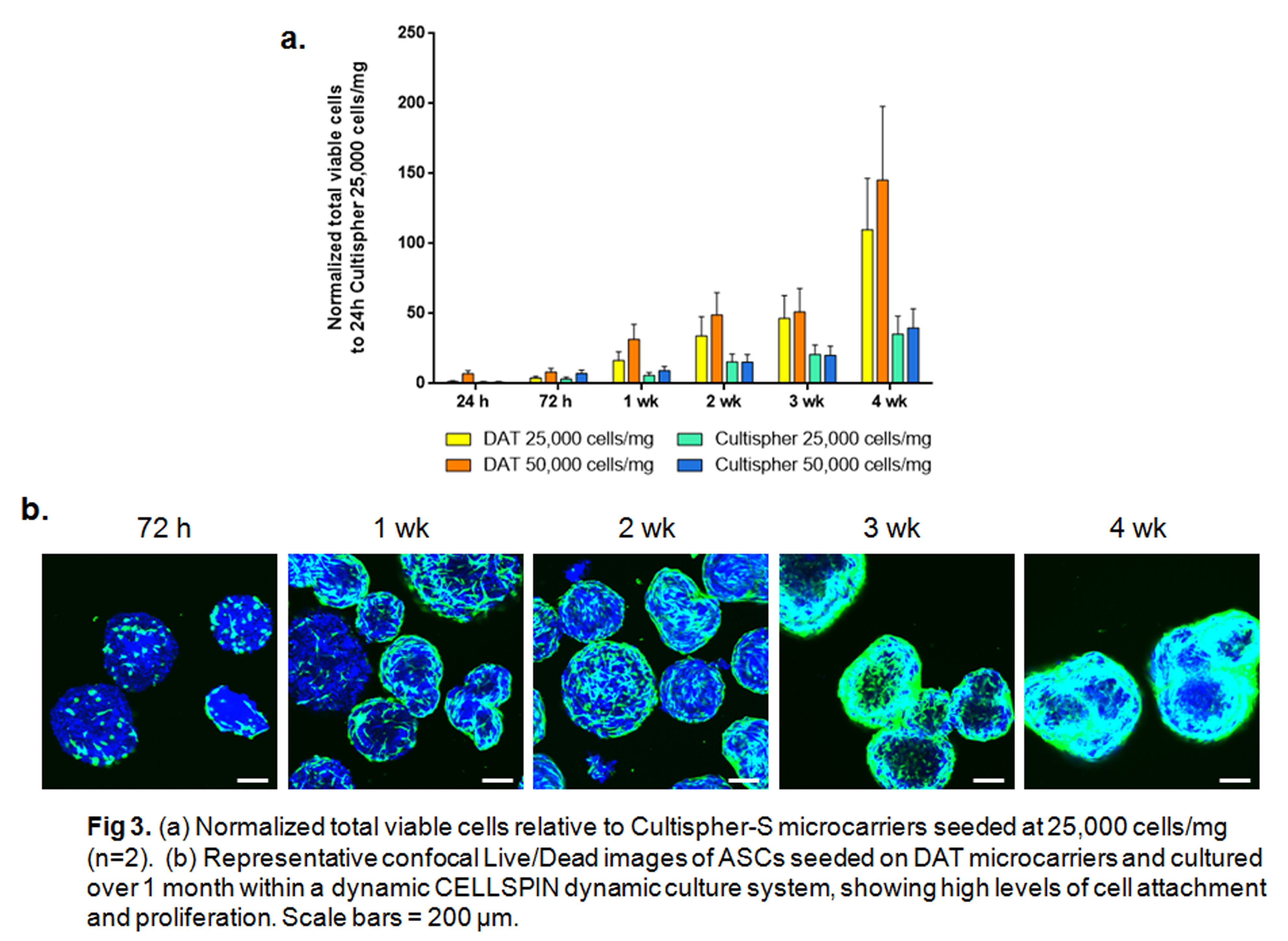
Conclusion: ECM-derived microcarriers hold promise as a clinically translatable strategy to regenerate a wide range of soft tissues and may be applied to advance current knowledge on how the tissue-specific ECM microenvironment influences cell behaviour.
Funding for this study was provided by the Natural Sciences and Engineering Research Council (NSERC) of Canada and the Canadian Institutes of Health Research (CIHR). The authors would like to thank Dr. B.G. Amsden for access to his high-voltage DC apparatus and Dr. J.F. Watkins and Mrs. K. Martin for their clinical collaborations.
References:
[1] Halper, J. & Kjaer, M. Basic components of connective tissues and extracellular matrix: elastin, fibrillin, fibulins, fibrinogen, fibronectin, laminin, tenascins and thrombospondins. Adv. Exp. Med. Biol. 802, 31–47 (2014).
[2] Yu, C. et al. Porous decellularized adipose tissue foams for soft tissue regeneration. Biomaterials 34, 3290–302 (2013).
[3] Reing, J. E. et al. The effects of processing methods upon mechanical and biologic properties of porcine dermal extracellular matrix scaffolds. Biomaterials 31, 8626–33 (2010).
[4] Wainwright, J. M. et al. Preparation of cardiac extracellular matrix from an intact porcine heart. Tissue Eng. Part C. Methods 16, 525–32 (2010).