Application of a flow evolution network model for stress-strain predictions in absorbable coronary stents
-
1
FDA/CDRH, Office of Science and Engineering Laboratories/ Division of Applied Mechanics, United States
-
2
Veryst Engineering, United States
Introduction: In order to address the adverse event of late stent thrombosis, absorbable polymers are being investigated as the stent platform material in coronary stents[1]. Coronary stents experience significant permanent deformation when loaded onto the catheter and expanded as well as during cyclic loading once implanted. Computational modeling is critical to the design and non-clinical testing of coronary stents because it enables prediction of the stress-strain distribution and estimation of fatigue safety performance[2]. However, computational modeling of fully absorbable coronary stents is significantly hampered by limited constitutive models to predict the post-yield and viscoelastic behavior of the material. In this study, we investigated the applicability of a Flow Evolution Network (FEN) model[3] developed for a common absorbable polymer to predict the mechanical response of a model coronary stent sub-unit.
Methods: Model coronary stent sub-units were laser cut with an ultraviolet laser from an injection molded sheet of poly(L-lactide), i.e., PLLA, based on a design previously used to study fatigue of nitinol stents[4]. Axial loading of the stent sub-units results in bending of the elbows similar to the motions experienced at classical v-struts in coronary stents (Figure 1). The sub-units were loaded in tension until failure while immersed in water at 37°C. A finite element model (Abaqus Standard 6.14-1) was created of the stent sub-unit based on quarter symmetry using three-dimensional 8 node brick elements with full integration (11,000 elements). The PLLA material was modeled with a FEN model[3] which is a micromechanism inspired constitutive model that accounts for large strain viscoplasticity and softening post-yield. Boundary conditions for the simulation mimicked the experiment.
Results and Discussion: The finite element simulation predicted that the critical stress location was at the elbows in the linear portion of the curve (Figure 1). As the specimens were loaded into the non-linear region of the curve, high stresses consistent with plastic deformation were also generated at the transition between the specimen arms or struts and the tabs which facilitated loading (Figure 1). As the specimen approached failure, Von Mises stresses and equivalent strains at the critical locations were consistent with values expected to produce failure in PLLA. The force-displacement curve predicted by the simulation compared well with experimental data (Figure 2), particularly in the linear and near-constant stress regions. These regions are most relevant to the types of loading and deformations that would be experienced by a stent in vivo. The results demonstrate the validity of using the Flow Evolution Network model for PLLA and its application to a coronary stent geometry and loading profile.
Conclusions: The Flow Evolution Network model is able to predict the mechanical behavior of PLLA when used for coronary stent design.
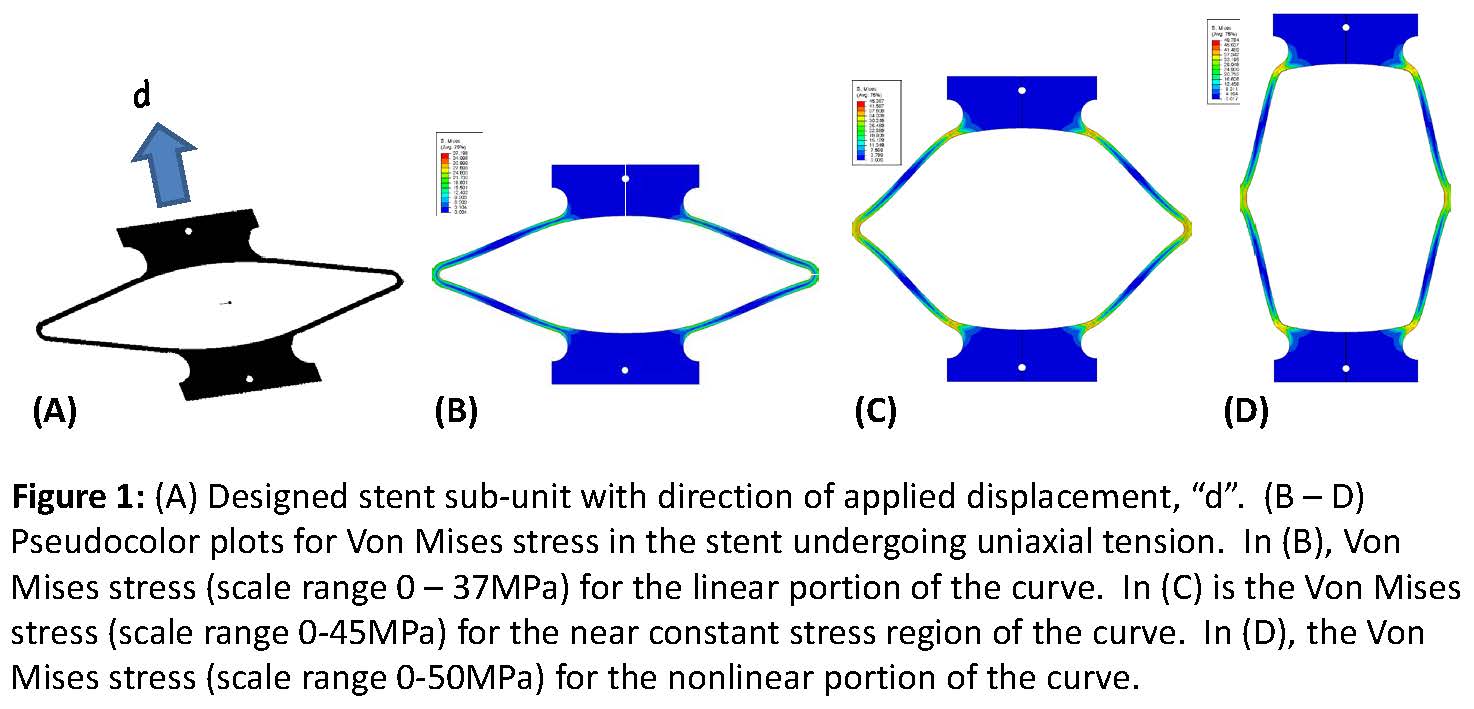
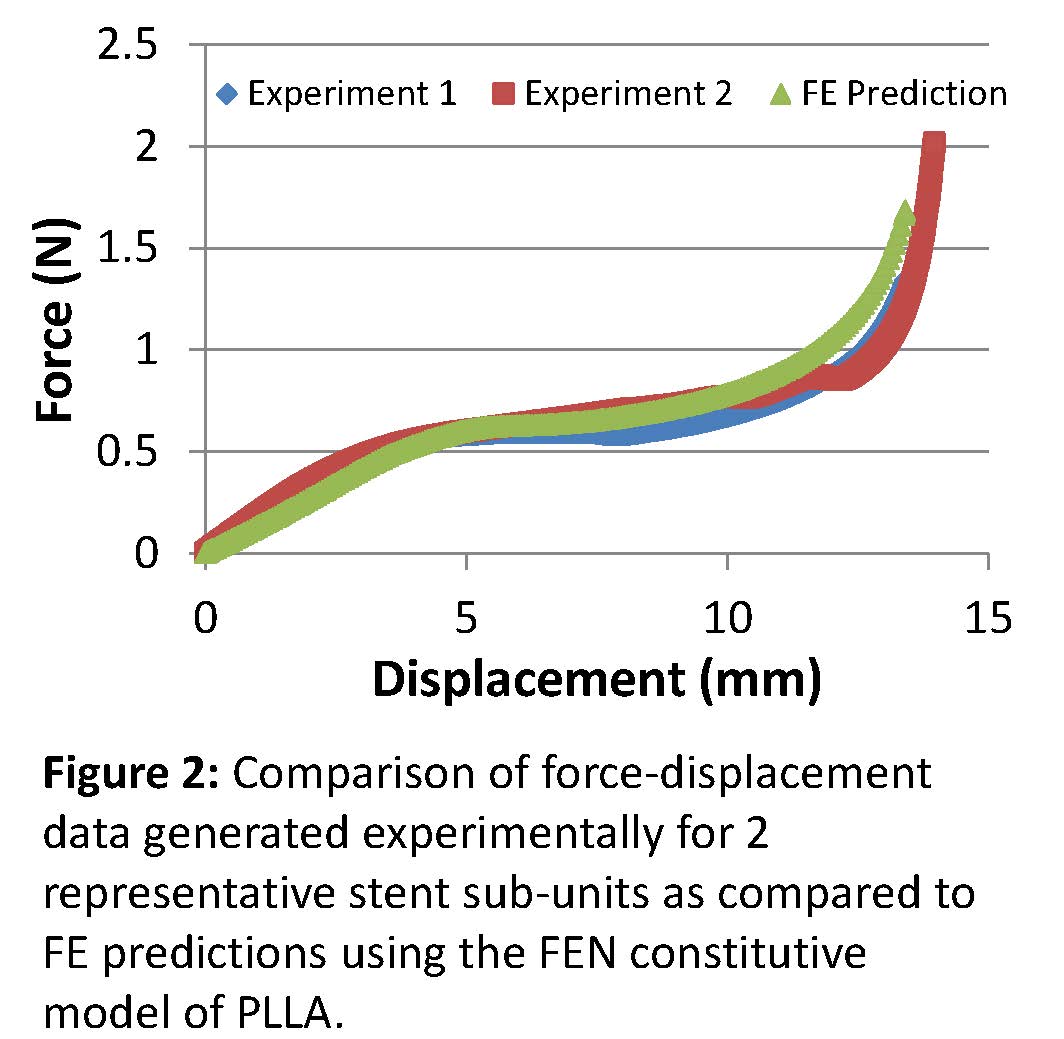
References:
[1] Serruys et al., 2012, European Heart Journal,33: 16-25.
[2] US Food and Drug Administration, CDRH., 2010. Guidance for Industry and FDA Staff: Non-Clinical Engineering Tests and Recommended Labeling for Intravascular Stents and Associated Delivery Systems.
[3] PolyUMod Software Library from Veryst Engineering, Needham, MA
[4] Pelton et al 2008, Journal of the Mechanical Behavior of Biomedical Materials, 1: 153-164.
Keywords:
modeling,
mechanical property,
Biodegradable material
Conference:
10th World Biomaterials Congress, Montréal, Canada, 17 May - 22 May, 2016.
Presentation Type:
General Session Oral
Topic:
Computational modeling in biomaterials science and engineering
Citation:
Dreher
M,
Nagaraja
S,
Bergstrom
J and
Hayman
D
(2016). Application of a flow evolution network model for stress-strain predictions in absorbable coronary stents.
Front. Bioeng. Biotechnol.
Conference Abstract:
10th World Biomaterials Congress.
doi: 10.3389/conf.FBIOE.2016.01.01974
Copyright:
The abstracts in this collection have not been subject to any Frontiers peer review or checks, and are not endorsed by Frontiers.
They are made available through the Frontiers publishing platform as a service to conference organizers and presenters.
The copyright in the individual abstracts is owned by the author of each abstract or his/her employer unless otherwise stated.
Each abstract, as well as the collection of abstracts, are published under a Creative Commons CC-BY 4.0 (attribution) licence (https://creativecommons.org/licenses/by/4.0/) and may thus be reproduced, translated, adapted and be the subject of derivative works provided the authors and Frontiers are attributed.
For Frontiers’ terms and conditions please see https://www.frontiersin.org/legal/terms-and-conditions.
Received:
27 Mar 2016;
Published Online:
30 Mar 2016.
*
Correspondence:
Dr. Maureen Dreher, FDA/CDRH, Office of Science and Engineering Laboratories/ Division of Applied Mechanics, Silver Spring, MD, United States, Email1
Dr. Srinidhi Nagaraja, FDA/CDRH, Office of Science and Engineering Laboratories/ Division of Applied Mechanics, Silver Spring, MD, United States, srinidhi.nagaraja@fda.hhs.gov
Dr. Jorgen Bergstrom, Veryst Engineering, Needham, MA, United States, jbergstrom@veryst.com
Dr. Danika Hayman, Veryst Engineering, Needham, MA, United States, dhayman@veryst.com